Dr Shurui Miao: Interaction Among Ions in Complex Electrolyte Solutions
2024 is on track to be the first year on record to experience global temperature exceeding 1.5 °C above the pre-industrial levels (WMO). Scientific reports have repeatedly pointed out that reducing greenhouse gas emissions alone is not enough to prevent climate disasters. We urgently need scalable and reliable carbon capture and storage technologies to accelerate the process of achieving global net zero and negative emissions.
The natural weathering process of volcanic rocks provides a promising pathway for rapid and secure carbon storage. During this process, rocks rich in essential ions react with water and carbon dioxide (underground) to form stable carbon minerals such as limestone. Under some physical conditions, 95% conversation is achieved within 2 years on the scale of 40,000 tonne/year (Carbfix). However, many fundamental scientific questions remain unclear and limit the widespread adaptation of this technology.
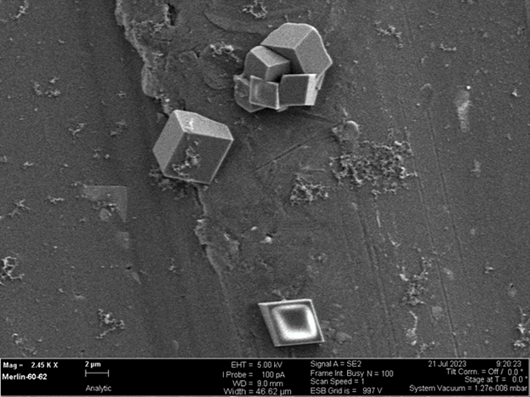
My work focuses on understanding the ion correlations and their roles in bulk solutions, at liquid-mineral interfaces, and during the formation of mineral particles. I use a range of physical chemistry experiments to study the distribution and interaction between electrical charges on the atomic length scale. I want to understand how naturally occurring physical conditions can affect the rate and sustainability of carbon mineralisation. Key parameters include the dimension of confinement (i.e. pore size of volcanic rocks), temperature and pressure (i.e. how deep underground is optimal), and salt concentration (i.e. in the presence of sea and underground water).
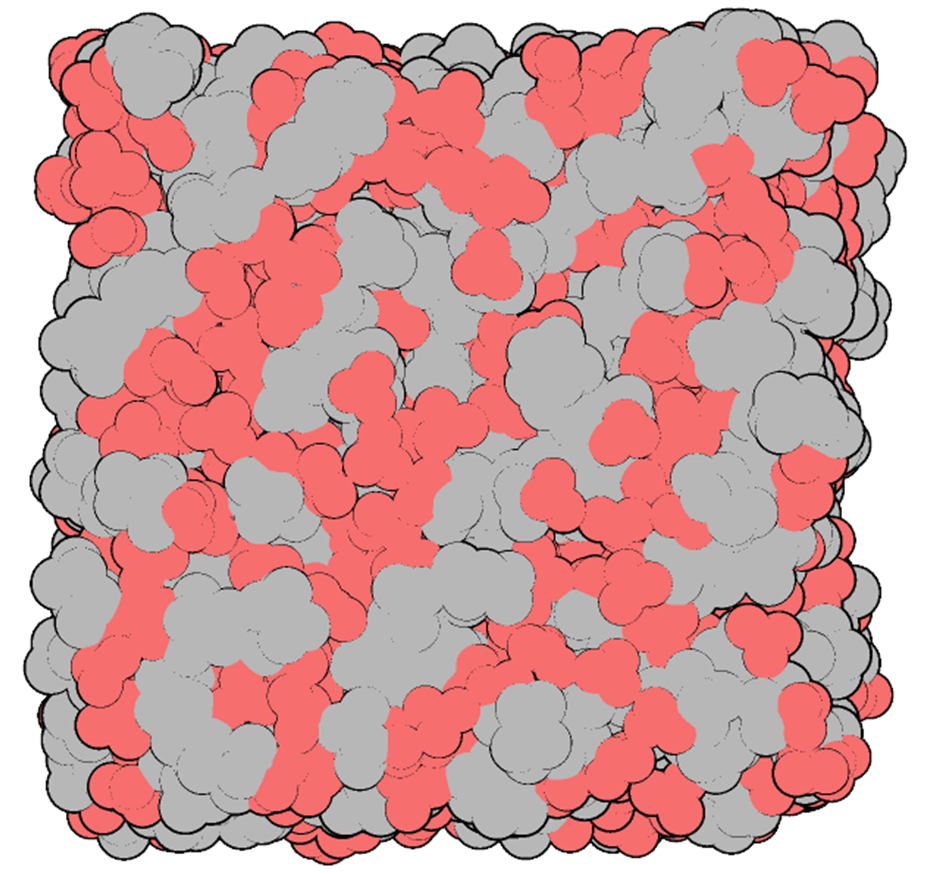
In addition to tackling global warming, I am also fascinated by our lack of fundamental understanding of concentrated electrolyte solutions. Despite how common these solutions are in our daily experiences (e.g. batteries and cellular fluids), we lack the theory to explain and predict their properties such as viscosity and conductivity. At sufficiently high concentrations, ions are close enough to interact and their motions become correlated. As a result, clusters and networks start to form (Bjerrum length > charge separation: lb>b+-; see diagram), resulting in collective behaviours that cannot be predicted by mean field theorems nor by studying individual ions alone.
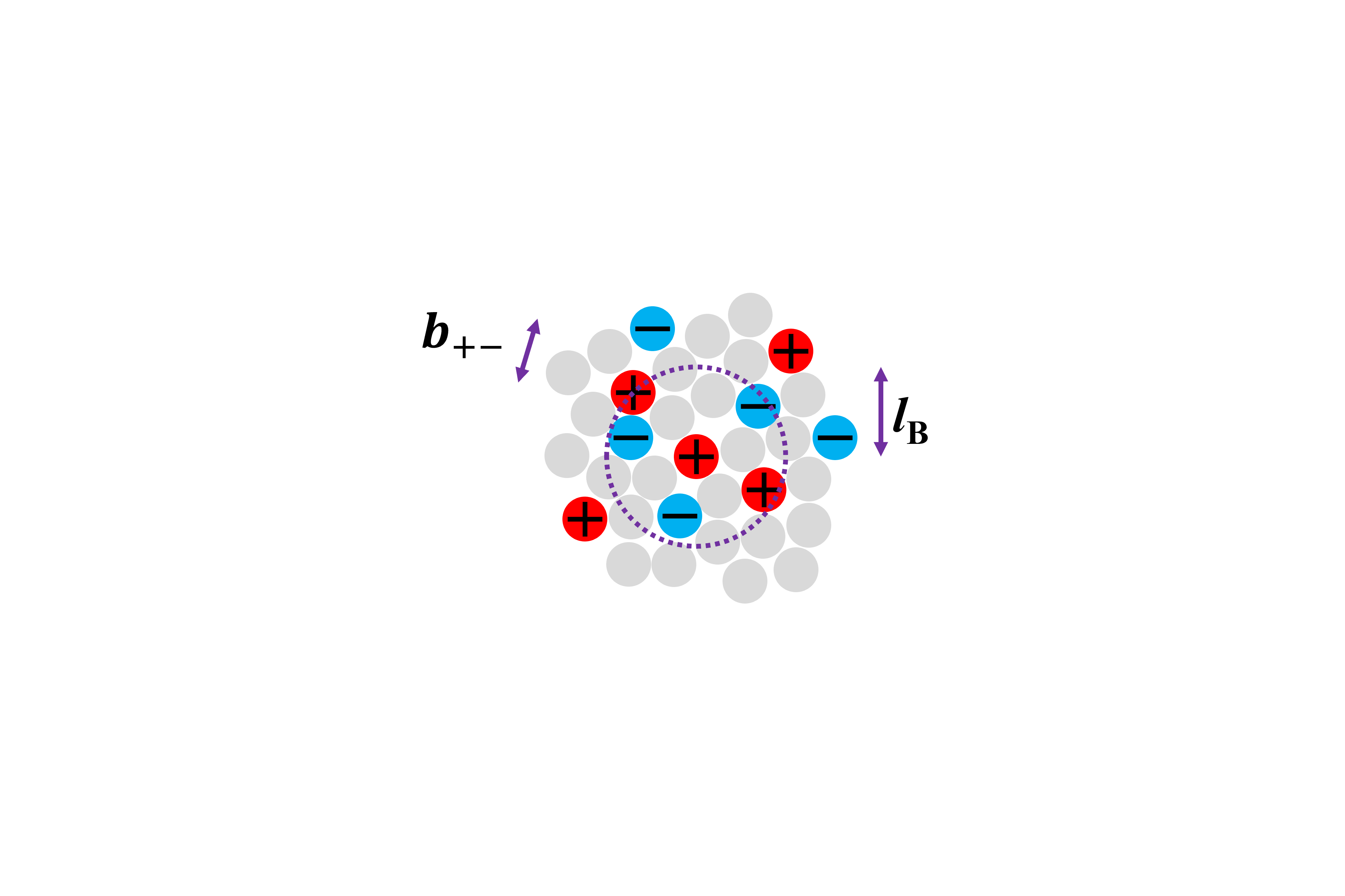
With rapidly advancing technologies, new solutions must emerge to meet the needs of society. The development of our understanding of solutions has transformed our view of nature, enabled the industrial production of chemicals, and to some extent reflects the development of chemistry itself. I am interested in employing the latest experimental techniques to understand the relationship between the structure, dynamics, and functionality of these disordered materials on the atomic scale.
If you would like to learn more about Dr Miao’s research, a full list of his publications is available via Google Scholar.
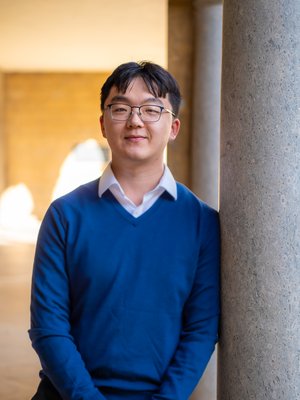